Bone is a really awesome material, being hard and flexible at the same time. For something so ubiquitous and studied for so long, it might come as a surprise to some of you to hear that the molecular bone structure of bone has alluded scientists for such a long while. This is because, even though the constituent elements that make up bone have been known for a long time, how they line together to form such an intricate structure has been very difficult to identify, until recently after MIT researchers used supercomputers to reveal with almost atomic precision the precise structure of bone. Their work is a significant step forward in material science, marking a milestone. The researchers hope they can synthesize bone fibers soon enough, while furthering their understanding of how some diseases attack bones.
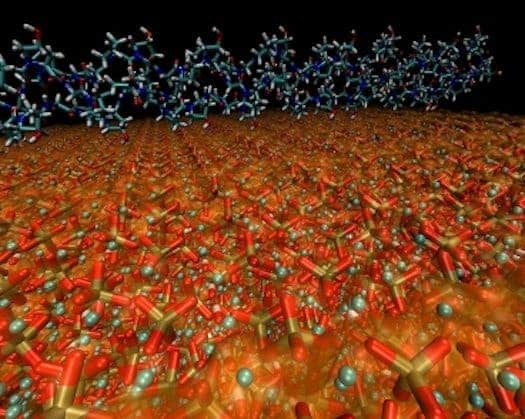
Rather curiously, bone is made out of two materials: a soft, flexible biomolecule called collagen and a hard, brittle form of the mineral apatite (hydroxyapatite). Each substance, taken individually, has nothing to do with bone, but when combined in a very complex manner, they form something that is simultaneously hard, tough and slightly flexible.
For years scientists have been studying bone at a molecular level, using tools such as atomic force microscopes to probe the material at an atomic level, however using such methods imaging is only possible in 2-D. Bone, taken as a whole, is an intricate molecular 3-D structure that can not be decoded by looking from a 2-D perspective.
“It’s easy to get images of bone, but it’s hard to see exactly where the minerals are located inside the collagen,” says Shu-Wei Chang, a CEE graduate student who was a co-author of the paper.
Using a supercomputer, engineers from MIT, led by civil engineer and materials scientist Markus Buehler, calculated what fibers of collagen, strengthened with hydroxyapatite crystals, look like. To verify their findings, they made a comparative study with laboratory findings. This whole process required several iterations before they could come to a sound conclusion and took several months to complete, something very important to note since the same process would have taken years and years to complete not too long ago. Thankfully supercomputing has evolved exponentially and MIT were quick to update their stock.
What makes up bones
According to their results, the MIT researchers found that the key to the bone’s fantastic molecular structure lies in how the hydroxyapatite crystals align with collagen. Hydroxyapatite grains are tiny, thin platelets just a few nanometers (billionths of a meter) across, and are deeply embedded in the collagen matrix. The two constituents are bound together by electrostatic interactions, which allows them to slip somewhat against each other without breaking. Large pieces of hydroxyapatite are brittle, like chalk, but at such small sizes, the mineral is actually ductile. The researchers also found that under stress tests, the mineral parts of the fibers took on four times as much stress as the collagen portions, whereas bending happened almost exclusively in the collagen.
“In this arrangement of tiny hydroxyapatite grains embedded in the collagen matrix, the two materials can each contribute the best of their properties,” Buehler says. “Hydroxyapatite takes most of the forces in the material, whereas collagen takes most of the stretching.”
Buehler and his team hope they can next create bone fibers in the lab. Also, now with a better understanding of what makes up bone and how its constituent elements are arranged, doctors may find what goes wrong in certain diseases, including osteoporosis and brittle bone disease.
“We can use this model to understand how a bone becomes more brittle,” says Arun Nair, a CEE postdoc who was the first author of the paper. For example, collagen is made up of thousands of amino acids, but “if only one of those amino acids is altered, it changes the way the minerals form” inside the bone, Nair says.
“That’s why this model is so critical,” he adds. Without it, you could observe how bone changes as a result of disease, but “you don’t know why. Now, we can see how a very tiny change … changes the way the mineral grows, or how the forces and deformation are distributed.”
The findings were reported in the journal Nature Communications.
Was this helpful?