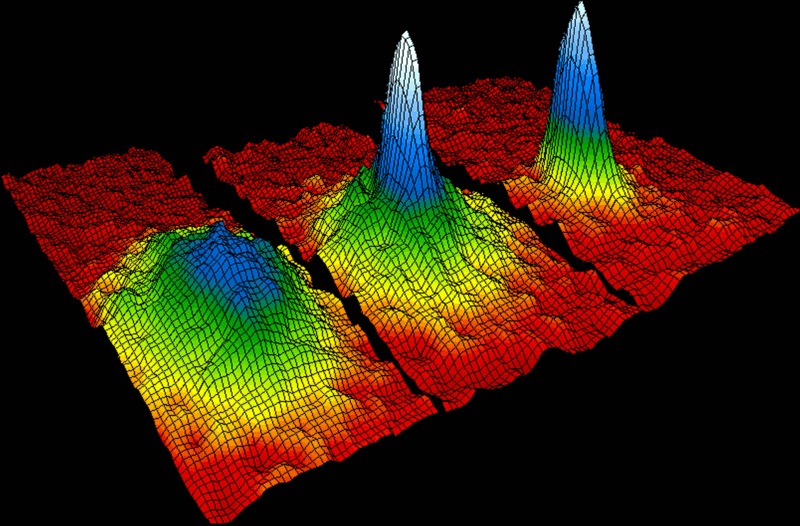
A team of physicists from the University of Bonn developed a totally new type of source of light, the so called Bose-Einstein condensate; the results will be published in the upcoming edition of Nature. They managed to achieve this astonishing feat by greatly cooling Rubidium atoms and stashing them into each other, up until the point they become indistinguishable and behave like a single big particle, which researchers call a Bose-Einstein condensate.
Technically speaking, the Bose-Einstein condensate is a state of matter of a dilute gas, of weakly interacting bosons, cooled to a temperature very close to absolute zero (approximately -273 degrees Celsius). Under such conditions, a large fraction of the bosons occupy the lowest quantum state of the external potential, at which point quantum effects become apparent on a macroscopic scale. [Wikipedia]
The apparently unsolvable problem appears when talking about light; the problem is that when photons are cooled down, they just disappear; however, Bonn physicists Jan Klärs, Julian Schmitt, Dr. Frank Vewinger, and Professor Dr. Martin Weitz succeeded where so many others failed.
For a better understanding of the phenomena, we should ask ourselves how warm light really is; for example, if you warm a tungsten filament (standard light bulb filament), it starts glowing, first red, then moves on to yellow and then, finally, blue(ish). It would seem that for every temperature there is a different colour, but the problem is that different metals glow in different colours, so a common starting unit had to be found. In order to achieve this, physicists created a theoretical model, the so called black body. The black body is an idealized object that absorbs all the electromagnetic radiation directed at it. So if you take this theoretical object and theoretically heat it, it will give a common ground for a corelation between light colour and temperature. But what happens when you cool it ?

If you would cool it, it will at some point stop radiating in the visible spectrum – it will only give out infrared photons which are invisible for the human eye. Also, as you cool it, the radiation intensity will decrease as the number of photons gets smaller (because photons disappear when cooled). The problem seems impossible – how do you lower the temperature of the photons without “killing” them ?
The Bonn researchers used a really inventive system, basically using two highly reflective mirrors and bouncing a beam back and forth between them. What happens is that when light hits the mirrors, the molecules in the mirror absorb the photons and then spit them back, and a whole number of interesting things happen during those collisions:
“During this process, the photons assumed the temperature of the fluid,” explained Professor Weitz. “They cooled each other off to room temperature this way, and they did it without getting lost in the process.”
This should especially please chip designers, because they use laser light for etching logic circuits into their semiconductor materials; just how small and fine these structures can be is limited by the wavelength of light – the smaller the better. A big wavelength is just like writing on a piece of paper with a big paintbrush. In time, this development will pave the way for more performant microchips, which will ultimately affect us all.
Was this helpful?