A new MIT-designed polymer can both put up a tough front or show its softer side and heal — its mood changes with the ambient light.

Image credits Felice Frankel.
Materials have come a long way. Far gone are the days when we only had stones for tools and pelts to dress with — now we have fancy things like plastics, glass, metals or concrete to shape our world with. We’re also much better at processing them with high precision, which took us from shaky beds and tough-spun tunics to comfy, modern beds and comfy, modern clothing.
However, for all our advances in material science, we’ve yet to develop something that’s resilient without requiring occasional repairs. Since mending is tedious, boring, and occasionally impossible (think of a satellite in orbit), one research team from MIT decided to develop a material that takes care of that problem.
Researchers have dabbled in self-healing materials before. These efforts haven’t yet seen wide-scale applications, but they’re not exactly ‘new’ either. However, self-healing materials tend to trade resilience for their ability to repair themselves. What this new research brings to the table is a material that can be both hardy and self-healing — I say ‘both’ but it’s more of a case of ‘either/or’.
Light healing
A material’s structural strength stems from the way its constituent atoms and molecules tie to one another — a characteristic known as a material’s ‘topology’. Self-healing materials need a laxer topology with more permissive bonds in order to ebb and flow into any damaged areas. However, that also makes the end material much less resilient — which is a product of a rigid, well-knit topology.
To work around the problem, the team designed a polymer that can actually change its own topology in response to light.
You can switch the material states back and forth, and in each of those states, the material acts as though it were a completely different material, even though it’s made of all the same components,” says Jeremiah Johnson, an associate professor of chemistry at MIT, and lead researcher of the project.
Topology is something that typically remains fixed after a material is formed. Or, if this topology is altered, it’s usually not via a reversible process. Rubber, for example, becomes brittle over time due to ozonation and oxidation (reactions with ozone and oxygen, respectively), which alter its topology.
To make the process reversible, the team worked with polymers attached to photosensitive molecules. This way, they could use light to activate and change the topology of the material.
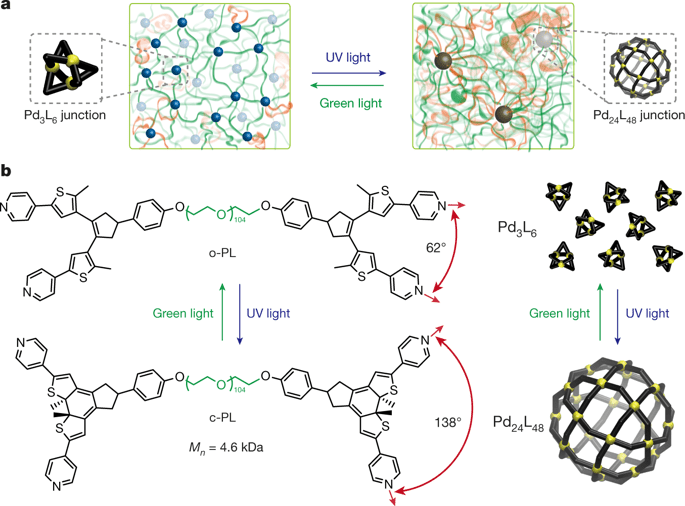
Image and caption credits: Yuwei Gu et al., 2018, Nature.
The team started from a class of materials they had designed previously, known as polymer metal-organic cages — or polyMOCs. The researchers describe them as metal-containing cage-like structures strewn together by flexible polymer bridges. The metal bits of the material are tied to the strands of polymer using chemical structures called ligands. Each metal atom (the team used palladium) can bind to four ligand molecules, and several of these sub-assemblies come together to create a rigid structure (the ‘cages’). The exact ratio of ligand molecules to metal atoms determines the size of the cages.
The team’s goal for the new material was to enable it to reversibly switch between two cage sizes: one with 24 atoms of palladium for 48 ligand molecules, and one with 3 palladium atoms for 6 ligand molecules.
Here’s where the photosensitive molecule DTE comes in. The ligand ties to the palladium atom via a nitrogen compound, and as most bonds in chemistry, this forms at a particular angle. The team mixed DTE into the ligand strands to act as a kind of light-operated switch that changes the angle of this bond.
When the material is exposed to ultraviolet (UV) light, this DTE molecule forms a ring and physically pushes the ligand strut away from the palladium — which alters the angle of the bond. This motion breaks apart the cages and forces them to re-form into larger ones.
By contrast, when the material is exposed to green light, that ring is broken, the ligands can tie to the palladium at a sharper angle, and the smaller cages re-form.
In the small-cage state, the material is up to 10 times softer and more dynamic than in the large-cage state, the team reports.
“They can flow when heated up, which means you could cut them and upon mild heating that damage will heal,” Johnson says.
Versatile, but short-lived
The switch takes around five hours to complete, and the team says their material can bear up to seven cycles before breaking down — with each reversal, some of the polymers fail to make the switch, which eventually results in local or wide-spread structural instability.
Still, for such an early stage, these are definitely exciting results. With some more refining, the material could serve in a wide range of applications — similar materials could coat objects such as cars or satellites, giving them a ‘skin’ that can heal damage.
“Anything made from plastic or rubber, if it could be healed when it was damaged, then it wouldn’t have to be thrown away. Maybe this approach would provide materials with longer life cycles,” Johnson adds.
Another potential application could include drug delivery. These could be encapsulated in the cages and released when exposed to UV light — then, upon exposure to green light, the same cages would recapture the substances, giving us a new way to control exactly where and when a drug is released in the body.
However, such applications are still far in the future. Apart from improving the number of cycles these materials can pass through to make them practical, the team also has to work on costs. They used polyethylene glycol (PEG) polymer, which isn’t very pricey and which should be replaceable by any kind of polymer. The real issue is the palladium, which is very rare and very expensive.
Another goal the team has set for the future is to create materials that can switch between a liquid and solid state using a similar system, and to use light to create softer and more solid sections within the same material.
The paper “Photoswitching topology in polymer networks with metal–organic cages as crosslinks” has been published in the journal Nature.
Was this helpful?