It lets you see or talk to a loved one in another corner of the world, and sometimes it fries you from outer space — it’s electromagnetic radiation and it’s a really nifty thing. So, let’s take a look at all the different kinds of electromagnetic radiation and why they all are, in fact, the same thing.

What Is Electromagnetic Radiation?
At its core, electromagnetic radiation (EMR) is a form of energy that travels through the vacuum of space at the speed of light. It is characterized by its wavelength and frequency, and it can be described by the electromagnetic spectrum, which is a way of organizing different types of electromagnetic radiation based on these properties.
The electromagnetic spectrum is a wide range of wavelengths and frequencies, and it includes everything from radio waves to gamma rays. Each type of electromagnetic radiation falls into a specific part of the spectrum, and each type has its own unique properties and uses.
“Waves are ubiquitous in nature,” writes physicist Shane Cloude, an expert on electromagnetic waves. “We are familiar with many of their physical properties from everyday experience. Waves on the surface of the water, and acoustic or sound waves, are all familiar phenomena.”
But electromagnetic waves are less obvious to our everyday experience, and there are several types electromagnetic radiation, such asfma:
- radio waves
- microwaves
- infrared radiation
- visible light
- ultraviolet radiation
- X-rays
- gamma rays
Type of Electromagnetic Radiation | Wavelength (m) | Frequency (Hz) | Uses |
---|---|---|---|
Radio Waves | 1 mm to 100 km | 3 kHz to 300 GHz | Television and radio broadcasting, cell phone communication, GPS navigation, medical imaging, detecting celestial objects |
Microwaves | 1 mm to 1 m | 300 MHz to 300 GHz | Cooking food, transmitting data, radar, medical treatments, studying material properties |
Infrared | 1 mm to 750 nm | 300 GHz to 430 THz | Thermal imaging, emitted by warm objects |
Visible Light | 750 nm to 400 nm | 430 THz to 750 THz | Seeing colors, communication, illumination |
Ultraviolet | 400 nm to 10 nm | 750 THz to 30 PHz | Sunburns, skin cancer, sterilization, curing materials |
X-Rays | 10 nm to 0.01 nm | 30 PHz to 30 EHz | Medical imaging, detecting abnormalities, killing cancer cells, studying material properties |
Gamma Rays | < 0.01 nm | > 30 EHz | Cancer treatment, studying celestial objects, security scanners |
At first glance, they can seem to be wildly different things. Like, X-rays can be used to peer through the skin, and ultraviolets give you a tan and a skin burn if you don’t use sunscreen. Totally different, right?
Well, not really.
Think of the electromagnetic radiation spectrum as a guitar string stretched over eight frets. Play the lowest note and you get radio waves, play the highest one and you get gamma rays. On a guitar, different vibrational patterns in the string will give off distinct sounds in the form of notes — our perception of them varies, but they’re all basically the same thing set on different intensity settings. Similarly, different oscillation patterns of magnetic and electrical fields will generate various kinds of EMR. We perceive them as completely different (some we can’t directly sense at all) but they’re all basically the same phenomena on different intensities.
A source generates EM radiation when there’s energy in the system because that’s what makes particles vibrate. As a rule of thumb, hotter bodies generate waves with more power and predominantly at higher frequencies. Frequency is measured in hertz (Hz), which is defined as one cycle per second. A frequency of one Hz means one wave is generated each second, one kHz means 1,000 waves are generated per second, and one GHz corresponds to one billion per second.

Image credits Richard F. Lyon / Wikipedia.
“The terms light, electromagnetic radiation, and radiation all rever to the same physical phenomenon: electromagnetic energy,” writes accomplished science writer Ginger Butcher. “This energy can be described by frequency, wavelength, or energy. All three are related mathematically such that if you know one, you can calculate the other two.”
Wavelength is equal to speed over frequency and is usually taken to represent the distance between two successive crests. Technically, however, it can be measured anywhere on the wave.
Lastly, electromagnetic radiation stands apart from the rest of electromagnetic phenomena in that they are ‘far-field’ effects. These waves aren’t limited to interacting with close-by objects, unlike the electrostatic effect, for example. Once generated, the waves can also hurtle through space (they ‘radiate,’ where the term ‘radiation’ comes from) without any more input from the charges that generated them. So these waves will keep going until they run out of energy — either because they hit some particles they can interact with, or because they simply fizzle out.
We’ve learned a lot about
So now we have a basic idea of how they form, cool. Let’s go through each type of electromagnetic radiation.
Radio waves

Image via Wikipedia.
Radio waves have the lowest frequencies of all types of EMR, and their photons carry the least amount of energy. Usually, anything between 3kHz and 300 GHz is considered to be a radio wave, although some definitions classify anything above 1 GHz or 3GHz as microwaves. This makes radio waves the sloths of EMR. Radio wave photons are spaced far apart — at 3khz, the wavelength is 100 km (62 mi) long and only 1mm (0.039 in) at 300 GHz — meaning they carry less energy than other types of ER.
Their interaction with matter is largely limited to creating a bunch of electric charges spread out over a lot of atoms — so each charge is pretty tiny. It’s useful, however, since this spreading allows a conductor tied to a circuit to transform radio waves back into some electrical signals. Couple that with their speed (all EM waves travel at the speed of light in a vacuum), and they’re really good for long-range communications.
Alternatively, if you have a conductor that isn’t tied to a circuit, say an airplane in flight, separation of those charges will generate new radio waves — this is what allows radar signals to ‘reflect’ off of stuff. The absorption or emission of radio waves always produces an electrical current, heat, or both.
Radio waves are used for a variety of purposes, including television and radio broadcasting, cell phone communication, and GPS navigation. They are also used in medical imaging and for detecting distant celestial objects, such as stars and galaxies.
Microwaves
Microwaves are electromagnetic radiation with frequencies between 300 MHz (wavelength 100 cm) and 300 GHz (0.1 cm). Apart from a bit more energetic photons and a shorter wavelength (which means more energy density), they’re kinda-radio-wave-ish really. In fact, microwaves are extensively used in communication as well, but with a few key differences from radio waves.
First is that you need a direct line of sight to the receiver, as microwaves don’t bend (diffract) around hills or mountains, they don’t reflect back from the ionosphere, or follow the planet’s curvature as surface waves. But they pack more of a punch than radio waves and can pierce through some of the things that radio can’t — like thick clouds or dust — due to their higher frequency.
Microwaves are used to transmit data over wireless networks, to communicate with satellites and spacecraft, in autonomous and classical vehicles for collision avoidance systems, some radio networks, keyless entry systems, and garage door remotes.
They’re also useful in ovens. The same process that allows radio wave absorption to generate heat makes a 2.45GHz (12cm) microwave very good at heating water. And since food always has at least some water, it means microwave ovens are a nifty way to heat up food.
Infrared radiation
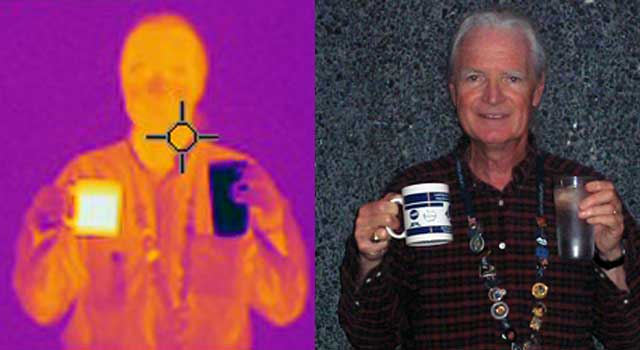
Image credits NASA / JPL.
The sweetheart of cheesy action movies, infrared, or IR. Its frequency spans from 300 GHz (1mm) to the lower visible limit (the color red) at 430 THz (700 nm). This is the spectrum over which most objects you’ll interact with radiate heat. Unlike radio and microwave radiation, infrared radiation interacts with dipoles (heavily polarized chemical molecules such as water), meaning it gets absorbed by a wide range of substances — and almost all organic substances — that turn its vibration into heat. However, the reverse is also true, meaning that bulk substances generally radiate some levels of IR as they release their heat.
So it’s not very good for long-range communications, since it would just get absorbed by the water in the atmosphere. But your TV remote can use IR to issue commands over short distances with great success. IR detectors are useful if you’re trying to see something that gives off heat — such as a burglar in the middle of the night. Infrared is also used in astronomy to peer through dust clouds in search of planets, in industrial applications to monitor for heat leaks or prevent overheating, in weather forecasting, and in certain medicinal applications. The military is also, obviously, a big fan of IR, using it both for observations and for guiding munitions toward a target.
And as lizard-lovers out there know, infrared radiation is a great way to beam heat where it’s needed. In fact, that’s exactly how people discovered IR. Back in 1800, an astronomer by the name of Sir William Herschel first described IR radiation by observing its effects on a thermometer.
Like all other electromagnetic radiation, IR carries energy and behaves both like a wave and like a quantum particle, the photon. A bit over half of all the solar energy that reaches Earth does so as infrared radiation — that’s why sunlight feels so warm.
Visible light
This is the interval of electromagnetic radiation that your eyes are tuned to pick up. Visible light spans the spectrum from 430-770 THz (390 to 700 nm). We see different colors because certain bits of this spectrum get absorbed by objects, and the rest gets reflected. For something to appear red to you, it needs to absorb the wavelengths that don’t correspond to the color and reflect just red wavelengths for your eyes to pick up.
However, color can also arise from the way light interacts with a particular object. An object’s texture is also created by much the same mechanism. Snow, for example, appears to be white, matte and reflective at the same time — but individual snow crystals look like bits of glass. You can find out why here.
Ultraviolet radiation

Image: Wikimedia Commons
The EM spectrum over the frequency of 789 terahertz (THz) or more is called ultraviolet. Ultraviolet light is composed of really short waves, from 10 nm to 400 nm, and carries a lot of energy. In fact, starting from the UV border, photons carry enough energy to alter certain chemical bonds into new arrangements. Which is hell if you’re a DNA molecule just trying to preserve information. Even worse for living stuff, certain UV subtypes that don’t have enough energy to damage DNA directly (such as subtype A) still pose a risk because they produce reactive oxygen species inside the body, highly reactive compounds that hijack chemical bonds in DNA.
Overall, UV radiation is energetic enough that it starts being a real hazard to life. Even relatively low-energy UV can cause nasty skin burns, far worse than those caused simply by heat (since they’re also radiation burns, as explained above). Exposure to higher-energy UV can lead to cancer, as the waves wreak havoc on DNA strands.
This ability to damage living organisms will be a common feature from now on the list, as frequencies will only keep increasing further on. At the higher ends of the UV spectrum (around 125 nm or less, sometimes called “extreme UV”), the energy carried by these waves is so high that it can actually strip electrons from atoms’ shells in a process called photoionization.
Considering that UV radiation constitutes about 10% of the sun’s total light output, it would cause a lot of trouble for anything living on land (since water does a pretty good job of absorbing UV). Luckily for us Earthlings, we’re protected by the ozone layer and the rest of the atmosphere, which filter out most UV rays before they cause any real damage.
It’s not all bad news, however. UV radiation is key to the synthesis of vitamin D in most land vertebrates, including humans. UV rays are also used in photography and astronomy, in certain security applications (to authenticate bills or credit cards), in forensics, as a sterilizer, and of course, on tanning beds.
X-rays / Röntgen radiation

With frequencies ranging from 30 petahertz to 30 exahertz (‘peta’ means 16 zeros, ‘exa’ means 19 zeros) and wavelengths from 0.01 to 10 nanometers, X-rays are very energetic. Those with wavelengths under 0.2–0.1 nm are called ‘hard’ X-rays. Doctors use them to see the bones inside the body because they’re so tiny and powerful that our soft tissues are virtually transparent to them. The same goes with luggage at the airport — hard X-rays can see right through them. Their wavelength is comparable to the size of individual atoms, which is why geologists use them to determine crystal structures.
X-rays (and the more energetic gamma rays) are made up of photons that all carry minimum-ionization energy (they can all photoionize), and are thus called ionizing radiation. They can inflict massive damage on organisms and biomolecules, often affecting tissues very deeply below the skin as they easily penetrate through most matter.
They are named after Wilhelm Röntgen, the German scientist who discovered them on November 8, 1895. Röntgen himself called them X-radiation because it was quite mysterious at the time — nobody really understood what this radiation was or what it did.
Gamma rays

Image credits NASA/Swift/Mary Pat Hrybyk-Keith and John Jones.
These are the EMRs with the single highest-energy photons we know of. They have frequencies in excess of 30 exahertz, and wavelengths of under 10 picometers (1 picometer is a thousandth of a nanometer or a thousandth of a billionth of a meter), which is less than the diameter of an atom. They mostly result from radioactive decay here on Earth (like nukes or Chornobyl), but can also come in ridiculously powerful gamma-ray bursts, likely the product of dying stars going supernova or the larger hypernova before collapsing into neutron stars or black holes. They are the single most deadly type of EM radiation for living organisms. Luckily, they’re largely absorbed by Earth’s atmosphere.
Artificial gamma rays are sometimes used to alter the appearance of gemstones, such as turning white topaz into blue topaz. The US is also experimenting with using them to create a sort-of X-ray machine on steroids that can scan up to 30 containers per hour. To get an idea of how ridiculously penetrative gamma rays are, know that mining operations use gamma-ray generators to look through huge piles of ore and select the richest for processing. Other uses include irradiation (used to sterilize medical equipment or foodstuffs), to kill cancer tumors, and in nuclear medicine.
In short, these are the categories we use to describe electromagnetic radiation. They have things they like to pass through, and things that they reflect from. They’re the light you can’t see and can be pleasant, very dangerous, and sometimes, insanely deadly.
We’re still learning something new about electromagnetic waves all the time. “Many advancements in electromagnetic theory were made in recent years,” writes Akira Ishimaru, a Japanese-American electrical engineer and professor emeritus at Department of Electrical and Computer Engineering at University of Washington.” No doubt, there is still much we’ve yet to learn about both the theory and the applications of this field of physics.
Was this helpful?