Researchers from the Goethe University in Frankfurt have refined our understanding of neutron stars by calculating the hard limit for their mass: these extreme stellar bodies cannot exceed 2.16 solar masses.
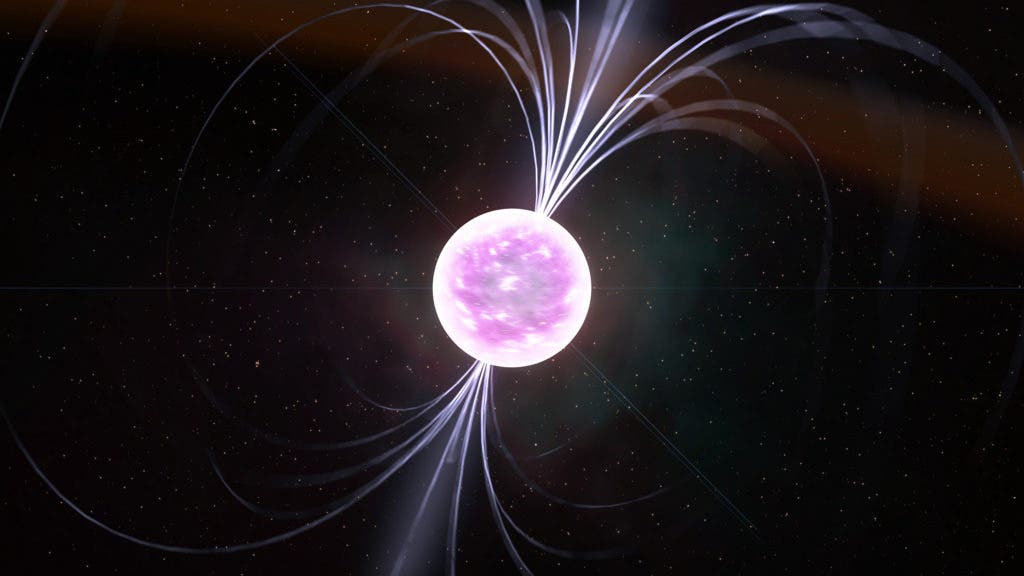
Neutron stars are one of the most extreme displays of matter around. They’re the naked cores of massive stars, compressed into pure matter in their death throes moments before a supernova detonates. Neutron stars aren’t made of regular atoms (which are over 99.999% empty space) rather they resemble one huge atomic nucleus. True to their name, neutron stars are incandescent bodies of neutron next to neutron.
In many ways, neutron stars are the closest matter can get to a black hole without collapsing space-time around it. Which also raises an interesting question — how massive can these stars actually become?
Weight-watching
With radiuses that generally fall under 12 kilometers (7.45 miles) but with masses that can be twice as great as that of our sun, neutron stars produce gravitational fields comparable to those of black holes. Unlike their black-hole brethren, however, neutron stars can’t grow indefinitely. Since they’re so immensely dense, there’s almost no force in nature that can withstand their gravitational force. So, the logic goes, if they become massive enough, that same gravitational pull will overcome the neutron’s ability to resist it. Going by that same train of thought, there should be a point beyond which even the addition of a single neutron will send the neutron star collapsing into a black hole.
Researchers have been trying to determine that exact point ever since neutron stars were first discovered in the 1960s — a question which they’ve only managed to answer now, as astrophysicists at the Goethe University Frankfurt have successfully calculated the strict upper limit for a neutron star’s maximum mass.
With an accuracy within a few percentage points, the maximum mass of non-rotating neutron stars cannot exceed 2.16 solar masses, the team reports.
The result was based on the “universal relations” approach developed in Frankfurt a few years ago. In broad strokes, these relations say that since all neutrons stars “look alike”, their properties can be expressed in terms of dimensionless quantities. The next piece of the puzzle was supplied by the LIGO experiment, in the form of data on the gravitational-wave signals and subsequent electromagnetic radiation discharge (kilonova) recorded last year during the merging of two neutron stars.
The LIGO data was instrumental in solving the problem as they allowed the team to decouple the calculations from the equation of state — a model we use to describe matter and its composition at various depths in a star.
“The beauty of theoretical research is that it can make predictions,” says Professor Luciano Rezzolla, the paper’s first author. “Theory, however, desperately needs experiments to narrow down some of its uncertainties.”
“It’s therefore quite remarkable that the observation of a single binary neutron star merger that occurred millions of light years away combined with the universal relations discovered through our theoretical work have allowed us to solve a riddle that has seen so much speculation in the past.”
The results were published in a Letter titled “Using Gravitational-wave Observations and Quasi-universal Relations to Constrain the Maximum Mass of Neutron Stars” in The Astrophysical Journal. They were confirmed a few days after publication by groups from the USA and Japan who followed different and independent approaches.