Heavier chemical elements could be the love child of two very spectacular and exotic lovers — neutron stars and tiny black holes.

Image via Digitaltrends.
We are, as Carl Sagan once put it, “made of star stuff.” Considering that what we call a star is actually a ginormous reactor mashing hydrogen and helium atoms into more complex elements, such as carbon, oxygen, or iron, that’s pretty true. But we needn’t look very hard around us for elements that outshine our particularly starry heritage. R-process elements (which are much heavier than iron — such as gold or uranium) could be sired by nature’s two most extreme creations.
Stellar birth
So let’s take a step back and look at how elements form. The lightest, simplest atom, hydrogen, was created during of the Big Bang, with some helium and traces of lithium and beryllium peppered in. This mix started to clump together in areas of higher density (accrete), which eventually led to the formation of the first stars. Stars that formed more complex elements. But there is a caveat. A living star simply isn’t powerful enough to fuse stuff past iron. Even supermassive stars, the biggest out there, can’t do it.
Think of a star as an explosion so massive, its sheer weight and gravitational pull causes it to fall back on itself. So a star can only exist while there’s a balance between two forces — the energy of fusion reactions trying to blast it apart, and gravity straining to keep it together. This works quite well for our granddaddy’os up to the point where they build up a respectable silicon core.
Because, as aging stars everywhere find out, fusing silicon into iron doesn’t return on investment. Even in the ultra-hot, uber-dense conditions of a star’s core, making atoms merge takes a lot of energy — needed to overcome protons’ (positively charged particles) tendency to push other positively charged particles away. However, once you do make them merge, protons and neutrons get along just great thanks to the nuclear force, which makes them stick together.
The simpler the atom, the stronger this nuclear force. So when you go from hydrogen (1 proton) to helium (2 protons), and then on to lithium (3 protons) it gets progressively weaker — it has ‘less power’ so to speak. At the same time, all that leftover power needs to go somewhere and it does so by degrading into the heat and light of stars. But there is a turning point where you end up needing to pump more energy into the atom to make it fuse than you get from its final nuclear force.
The flip-side is that this turning point works both ways — that’s why fusion reactors work with hydrogen but fission reactors work with uranium. You can extract the extra energy by lowering the nuclear force of simple elements (hydrogen), or you can get it by splitting heavier atoms (uranium) and cashing back on the energy it took to fuse them together. But that’s a story for another time.
What matters right now is that this turning point is iron.
Ms and Mr Dense
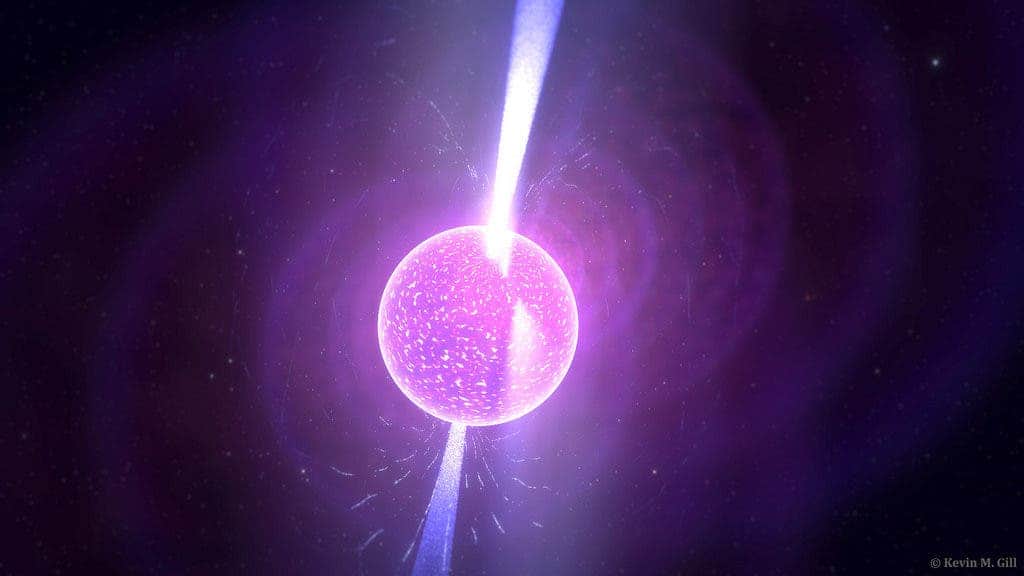
Image credits Kevin Gill / Flickr.
Up to now, supernovas and binary star mergers were believed to be the only environments that could supply the conditions needed for higher fusion. But now, a team of three theoretical astrophysicists at UCLA — George Fuller, Alex Kusenko and Volodymyr Takhistov — offer another event that could produce these elements: the merger between a tiny black hole and a neutron star.
“A different kind of furnace was needed to forge gold, platinum, uranium and most other elements heavier than iron,” Fuller, a theoretical astrophysicist and professor of physics who directs UC San Diego’s Center for Astrophysics and Space Sciences and first author of the paper, explained in a statement.
“These elements most likely formed in an environment rich with neutrons.”
Neutron stars are immensely dense. They’re what’s left in the wake of stellar collapses and supernovae, a kernel of ultra-packed matter. A ‘normal’ atom has a nucleus, an electron shell, and a lot of empty space between the two. Neutron stars are like a huge atomic nucleus, made of back-to-back neutrons held together by gravity. To get an idea of what “immensely dense” means, it’s estimated that a spoonful of the stuff neutron stars’ surfaces are made of weighs about three billion tons.
The other half of this lovely couple is even denser — a tiny black hole, weighing between 10-14 and 10-8 solar masses. Unlike neutron stars, we’re not really sure that they really exist. But a lot of researchers (including Stephen Hawking) do believe they’re out there, a byproduct of the Big Bang, and could make up part of the dark matter — which has been proven to exist. If these micro black holes follow the distribution of dark matter in space, they’ll often co-exist with neutron stars. And this, the team argues, sets the stage for heavier elements to form.
Their calculations show that if a neutron star captures such a black hole and get devoured from the inside out by it, some of the dense neutron star matter can get thrown out into space by the ferocity of the event. Even a tiny bit of this matter is enough to seed the formation of a huge quantity of heavy elements, since it’s so dense.
“As the neutron stars are devoured,” Fuller explains, “they spin up and eject cold neutron matter, which decompresses, heats up and make these elements.”
“In the last milliseconds of the neutron star’s demise, the amount of ejected neutron-rich material is sufficient to explain the observed abundances of heavy elements.”
The team’s theory is especially intriguing since it also helps explain a few other unanswered questions about the universe. For example, it explains why there aren’t that many neutron stars in the galactic core, where there are a lot of black holes to hunt them down. Even more, the team says that the ejection of nuclear matter from tiny black holes chowing on neutron stars would explain three mysterious astronomical phenomena.
“They are a distinctive display of infrared light [‘kilonovas’], a radio emission that may explain the mysterious Fast Radio Bursts from unknown sources deep in the cosmos, and the positrons detected in the galactic center by X-ray observations,” Fuller concludes.
“Each of these represent long-standing mysteries. It is indeed surprising that the solutions of these seemingly unrelated phenomena may be connected with the violent end of neutron stars at the hands of tiny black holes.”
The paper “Primordial Black Holes and r-Process Nucleosynthesis” has been published in the preprint archive arXiv.
Was this helpful?