July 12th, 2012, marked a monumental day in the history of physics. At CERN in Geneva operators of the Large Hadron Collider (LHC) announced the discovery of a particle called the ‘Higgs boson.’
First theorised in the 1960s by Peter Higgs, François Englert, and Robert Brout, finding this particle led to a wealth of implications that changed the face of physics–changes that are still being felt ten years on.
Not only did the revelation of the Higgs boson complete what physicists call the Standard Model of particle physics–the best description we have of particles and interactions of a sub-atomic level–but, it also opened investigations into physics beyond this model.
It Ain’t Heavy…
The discovery of the Higgs boson confirmed to physicists the mechanism that gives most particles their mass. This is because the Higgs boson is a particle that arises from a Universe-spanning field called the Higgs field.
Additionally, just as the photon is a boson–a force-carrying particle–that arises from an electromagnetic field and comes into play when particles interact, the Higgs carries the mechanism by which the Higgs field grants mass.
One might not immediately see why the origin of mass is something that physicists ever needed to explain, but the origin of this problem goes back to when physicists first started to use quantum field theory (QFT) to understand one of the Universe’s four fundamental forces.
QFT had already cracked electromagnetism, but when the theory was applied to the weak nuclear force–which determines how quarks, particles that make up protons and neutrons, change flavour and can cause atoms to decay–a problem arose.
The analysis of the weak force with QFT suggested that to be mathematically consistent, its force-carrying particles the W and Z bosons should be massless–no problem initially as photons, the most famous boson arguably, are massless. But the fact the weak force is so strong over tiny subatomic distances and yet so weak over large distances demanded these bosons have mass.
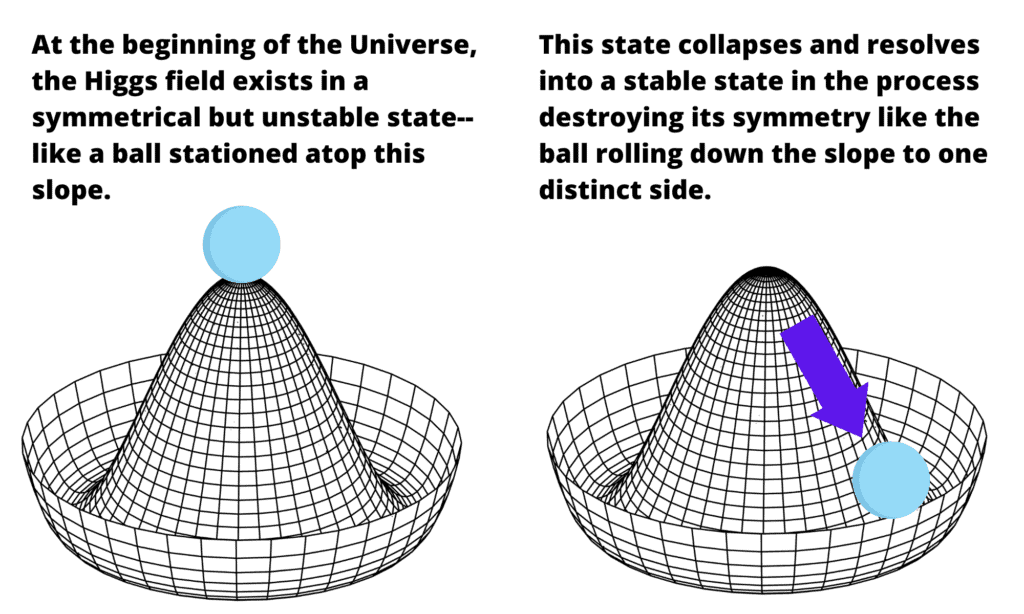
Physicists Peter Higgs, François Englert and Robert Brout had a suggestion. They posited a universal field that emerged at the beginning of the Universe. This field was born symmetrical but unstable.
As a common analogy for this think of a ball placed at the top of a perfectly symmetrical hill. The ball is in a symmetrical state–its faces in all directions equally. But the system is unstable and it must collapse into a stable state which it does by the roll rolling down the slope to one side–not the same in all directions–definitely unsymmetrical.
During this collapse–fractions of a second after the Big Bang– the Higgs field via the Brout-Englert-Higgs mechanism carried by the Higgs boson granted the W and Z bosons their mass.
On July 4th, ten years ago, by discovering the Higgs boson, CERN also provided experimental evidence to support this theory. But, more was to come. Like why should the Higgs field stop its mass-granting spree at the W and Z bosons? Why not all particles?
After the Discovery of the Higgs Boson
The first step after the discovery of the Higgs boson was to check if the Compact Muon Solenoid (CMS) and Atlas detectors at the LHC had actually detected the correct particle.
Because Higgs bosons only exist at extremely high energies similar to those that existed at the earliest moments of the Universe to detect them here on Earth today it takes an incredibly powerful particle accelerator that can slam together protons at speeds approaching the speed of light to create them.
Though the LHC is clearly up to the task, even then the Higgs boson created lasts for an immeasurably small amount of time–in turn making detecting them directly impossible.
Instead, researchers look for the cascade of secondary particles created when the Higgs boson decays. By March 2013, they had spotted enough of these cascades to confirm that the Higgs boson had indeed been found.
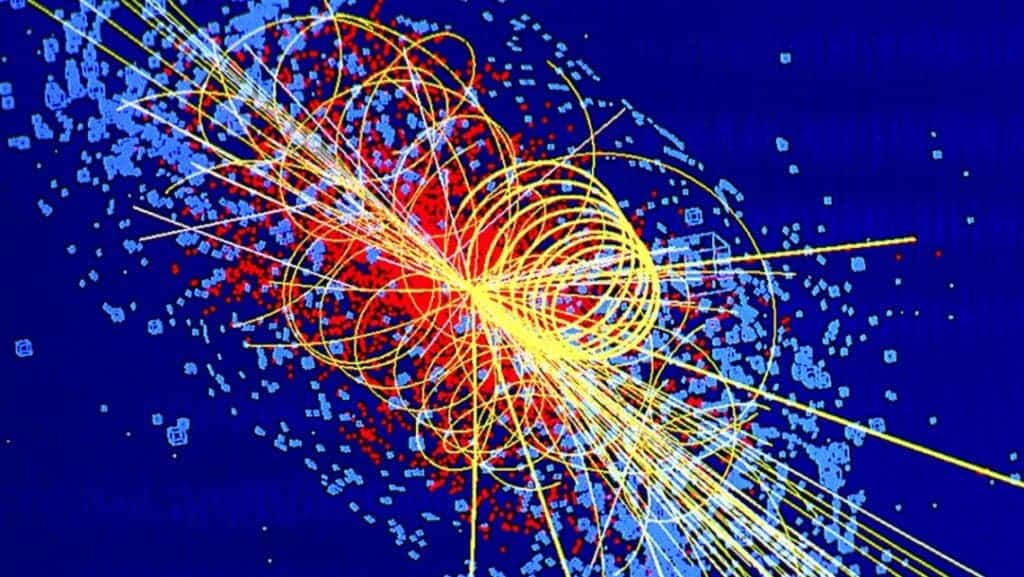
Following this, the quest began to discover if the Higgs boson also grants mass to other particles.
From what researchers have discovered since the Higgs boson was found, we now know that the “heaviness” of a particle depends on how strongly it interacts with the Higgs field.
So the top quark–one of the heaviest inhabitants of the particle zoo–gets its mass from a strong coupling with the Higgs field, something that thanks to investigations following 2012 physicists now understand quite well.
The weak coupling experienced by a lighter particle–the muon–is currently not as well understood, but physicists are now on their way to a better understanding of this.
The Higgs boson doesn’t just grant other particles mass, the boson also interacts with itself to grant its own mass.
What is Left to Discover About the Higgs boson?
One of the biggest mysteries that remain surrounding the Higgs boson is why and how it couples with the Higgs field to grant itself mass?
Answering this question could help explain why the universe experienced an imbalance between matter and antimatter during its earliest moments. An imbalance that resulted in the creation of all matter–if matter and antimatter existed in equal amounts then they would have annihilated each other before large-scale structures like stars and galaxies had the opportunity to form.
To understand how the Higgs boson interacts with itself, researchers at CERN are on the hunt for Higgs boson pairs and triplets–things that can only exist if it self-couples.
Current predictions suggest that Higgs couples are about 1,000 times rarer than single Higgs particles, and triplets are even rarer.
Fortunately, the high-luminosity upgrade the LHC has been undergoing will enable it to conduct more collisions and thus create more Higgs bosons–thus improving the odds of spotting a rare pairing or elusive tripling of the particle.
For a comparison of before and after Higgs production, after 2029 –the year the high luminosity LHC is expected to come online–LHC should create 15 million Higgs bosons per year. In 2017, it produced just 3 million of these force-carrying particles.
Beyond the Standard Model: Meet the Higgs Family
Theories that extend beyond the Standard Model predict that there could be as many as five different types of Higgs boson particles. This means that the search is on for relatives of the boson predicted by the Standard Model.
The Higgs boson discovered in 2o12 has no electric charge and is also the only inhabitant of the particle zoo to have zero spin–a quantum mechanical version of angular momentum that determines how a particle behaves when it encounters a magnetic field.
Researchers want to know if there are Higgs versions with different properties. Some models of physics beyond the standard model even hint that there may be one type of Higgs boson that interacts with heavy particles like the top quark, and another that grants mass to lighter particles like the muon.
One of the biggest hints of physics beyond the Standard Model is the existence of dark matter. This mysterious substance is responsible for over 80 per cent of the Universe’s mass (though when considering matter and energy this contribution is still dwarfed by dark energy) and its interactions with gravity literally prevents galaxies from flying apart as they spin.
What dark matter particles don’t do is interact with light–electromagnetic radiation–or with other particles of matter that compose everything we see around us on a day-to-day basis–baryonic matter.
But, due to interactions with gravity, we know that dark matter has mass, and it stands to reason these particles got this mass the same way as all the others, barring photons which don’t interact with the Higgs field, did–through interactions with the Higgs field enabled by the Higgs boson. Thus, ordinary matter should interact with dark matter via these force-carrying particles and there should be a detectable dark matter trace in the decay of Higgs boson particles.
That means these particles which took five decades and the largest, most expensive experiment in human history to detect, could be key in unlocking the dark matter mystery.
It’s little wonder that the announcement of the discovery of the Higgs boson on July 4th, 2012, in a lecture hall at CERN moved many physicists to tears, including Peter Higgs himself. It was really just the beginning of amazing discoveries to come.
Here’s to the next decade of the Higgs boson!
Was this helpful?