Stanford chemical engineers have developed a theoretical model that sheds light on the electrical conductivity properties of polymers. Their work provides a valuable first step for other researchers to build on, providing an experimental setting for those looking to expand the electrical conductivity of certain polymers (typically plastics) for use in the industry.
The word “polymer” is derived from the Greek for “many parts” which aptly describes their simple molecular structure, which consists of identical units, called monomers, that string together, end to end, like so many sausages. Humans have long used natural polymers such as silk and wool, while newer industrial processes have adapted this same technique to turn end-to-end chains of hydrocarbon molecules, ultimately derived from petroleum byproducts, into plastics.
The constitutive elements that go in your typical electronics like your smartphone or notebook include things like circuitry, transistors, condensers and so on, all typically made out of metallic materials, since these need to be electrically conductive. At the same time, however, these materials, like the reigning king silicon, are brittle and fairly stiff.
Fad or not, in recent years scientists have made various attempts at developing electronics capable of being stretched a significant or even multiple times their width, as well rolling. Imagine clothing electronics, tablets that can fold like a newspaper, a whole range of new possibilities. As such, many have experimented with polymers which are more flexible. It’s clear that there’s a serious trade-off problem here that engineers need to tackle: metals can’t stretch but they conduct electricity better, polymers can stretch, but conduct electricity poorly. Things don’t need to be all black and white, though. The best and quickest solutions are found when engineers have access to as much data and information about the problem they’re trying to solve as possible.
Stanford chemical engineering professor Andrew Spakowitz and colleagues the first theoretical framework that includes the molecular-level structural inhomogeneity of polymers. Metals have a regular molecular structure that allows electrical current to flow smoothly, but this is also what makes them rigid. Polymers on the other hand, at a molecular level, look more like a bowl of spaghetti: strands are coiled or run relatively true, even if curved, like lanes on a highway. This variability of molecular structure is reflected in the variability of electrical conductivity as well. In the process of experimenting with polymeric semiconductors, researchers discovered that these flexible materials exhibited “anomalous transport behavior” or, simply put, variability in the speed at which electrons flowed through the system.
“Prior theories of electrical flow in polymeric semiconductors are largely extrapolated from our understanding of metals and inorganic semiconductors like silicon,” Spakowitz said, adding that he and his collaborators began by taking a molecular-level view of the electron transport issue.
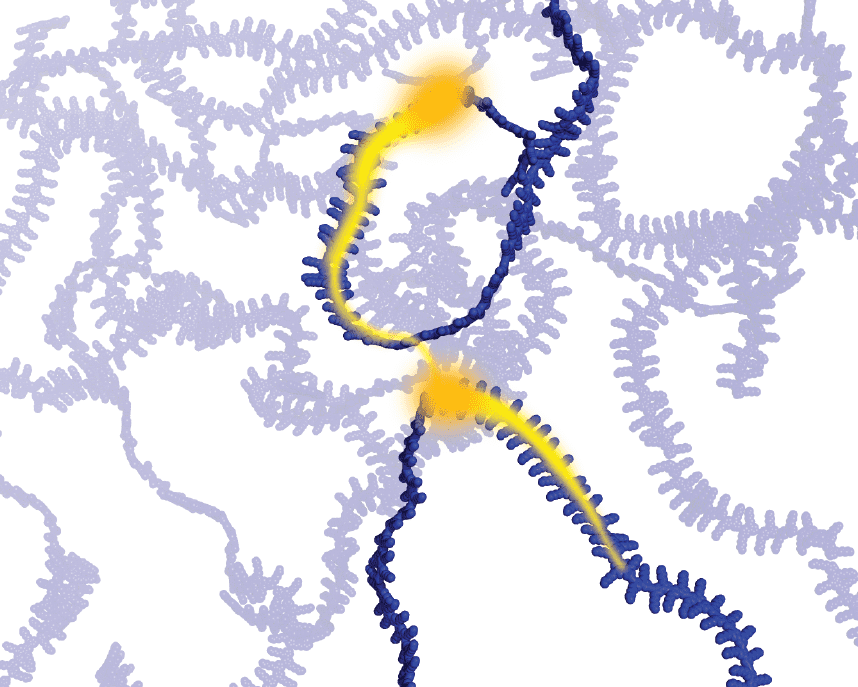
This insight is fundamental to future experiments and research dwelling into building stretchy electronics. One other important hallmark of the Stanford scientists’ paper is that they provide a simple algorithm that begins to suggest how to control the process for making polymers, with an emphasis on how manipulating their electrical conductivity properties.
“There are many, many types of monomers and many variables in the process,” Spakowitz said. The model presented by the Stanford team simplifies this problem greatly by reducing it to a small number of variables describing the structural and electronic properties of semiconducting polymers.
“A simple theory that works is a good start,” said Spakowitz, who envisions much work ahead to bring bending smart phones and folding e-readers to reality.
The author’s theory was published recently in the journal Proceedings of the National Academy of Sciences.
Was this helpful?