A group of researchers at MIT have devised a new method for infiltrating cells with large molecules such as nanoparticles or proteins that is a lot more non-intrusive and doesn’t damage the cell. Imaging target cells or growing more stable stem cells might thus be possible with this method.
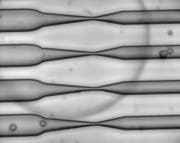
Image: Armon Sharei and Emily Jackson
Every cell has a membrane, which is put to great use as it protects the cell’s inner environment by regulating what gets in and what gets out. Typically, you don’t want foreign molecules entering your cells, but sometimes you do. Various methods have been employed to breach cell membranes and introduce other bodies, however these tend to be intrusive and sometimes can lead to the damaging and even destruction of the cell.
The MIT method of introducing large molecules in cell is a lot safer and efficient and implies squeezing the cell through a narrow construction just enough for tiny, yet temporary, gaps to surface. Prior to squeezing the cell, large molecules – be it RNA, proteins or nanoparticles – are tasked to float outside cell, such that when the holes pop these slide through the membrane instantly.
Through this technique the MIT researchers were able to deliver reprogramming proteins which turned the target cells into pluripotent stem cells – notoriously difficult to generate efficiently – with a success rate 10 to 100 times better than any other existing method. A simply massive advancement. Also, they’ve also tested the method with other large molecules like special nanoparticles, like carbon nanotubes or quantum dots, to image cells and thus monitor their activity.
“It’s very useful to be able to get large molecules into cells. We thought it might be interesting if you could have a relatively simple system that could deliver many different compounds,” says Klavs Jensen, the Warren K. Lewis Professor of Chemical Engineering, professor of materials science and engineering, and a senior author of a paper describing the new device in this week’s issue of the Proceedings of the National Academy of Sciences.
The team’s fantastic research builds upon previous work, when Jensen and Robert Langer, the David H. Koch Institute Professor at MIT and also a study lead author, forced molecules into cells as they flowed through a microfluidic device. The process was slow and not very effective, but it was during this time that the researchers learned that if you squeeze a cell just right now, tiny holes will appear – pure windows of opportunity.
Capitalizing on this, the scientists then proceeded to adjust their set-up and devised some rectangular microfluidic chips, no larger than a quarter, fitted with 40 to 70 parallel channels. Cells are suspended in a solution with the material to be delivered and flowed through the channel at high speed — about one meter per second. Halfway through the channel, the cells pass through a constriction about 30 to 80 percent smaller than the cells’ diameter. The cells don’t suffer any irreparable damage, and they maintain their normal functions after the treatment.
“This appears to be a very broadly applicable approach for loading a diversity of different compounds into a diversity of different cells,” says Mark Prausnitz, a professor of chemical and biomolecular engineering at Georgia Tech, who was not part of the research team. “It’s a really nice example of taking devices from the world of engineering and microelectronics and using them in quite different ways to solve problems in medicine that could have really great impact.”
source: MIT
Was this helpful?