
All atoms are made of three subatomic particles: the proton, the neutron, and the electron. Because the negatively charged electrons are naturally attracted to the positively charged nucleus, which is made of neutrons and protons, they perform an orbiting motion around the nucleus.
Does that sound familiar? It’s really no coincidence that many atomic pictures or chemistry 101 classes depict the components of various atoms like solar systems. The nucleus of the atom is the sun, and the electrons are the planets performing an orbiting motion around their parent body.
This solar system model of the atom is easy to understand and good enough for illustrating the most basic principles of chemistry. However, this model is quite wrong.
In reality, atoms don’t look or behave anything like the solar system. Planets are localized and follow precise orbits around stars governed by the laws of gravity.
In contrast, the motion of electrons is predicated on quantum mechanics, which means we can never know their exact locations or trajectories. Instead, electrons exist around the atomic nucleus in a probability ‘cloud’.
What is an electron cloud?
At the most basic level, an electron cloud is a region of space around an atom’s nucleus where electrons are likely to be found. This cloud is a fundamental aspect of the way that atoms are structured, playing a crucial role in determining the properties of different chemical elements.
To really understand the electron cloud, it’s important to first have a basic understanding of the nature of electrons. Electrons are subatomic particles with a negative electrical charge that orbit the nucleus of the atom. They are extremely light, with a mass that is over 1,000 times smaller than that of a proton. Despite their small size, electrons are incredibly important, as they are responsible for many of the chemical reactions that take place in the world around us.
The electron cloud is a representation of the probability of finding an electron in a particular region of space. This may sound odd at first since we just described the electron as a particle, a tiny bit of matter that should be found in a particular location in time and space. But that’s not at all how it works in the counter-intuitive and wacky world of quantum mechanics in which an electron’s true position can never be known with absolute certainty, so it follows that electrons orbiting the nucleus can be described as a cloud-like region of probability.
The density of this electron cloud is proportional to the probability of an electron being there, so the electron cloud will be its densest close to the nucleus. Meanwhile, the edges of the electron cloud will be fuzzy, indicating that electrons are far less likely to be in this zone, but are not necessarily absent.
From puddings to clouds: the winding road towards a quantum model of the electron
The notion of the atom can be traced back to more than 2,500 years ago in ancient Greece, when Democritus suggested that all matter in the universe was made up of tiny, indivisible, solid objects he called ‘atomos’. However, it wasn’t until the 19th century that this rather metaphysical model of the universe was formalized into a scientific framework after British chemist John Dalton performed experiments that provided evidence for atoms.
Dalton studied the pressure of gases and reckoned they must be made of tiny particles invisible to the naked eye that are in constant motion. Dalton’s research led to the development of a theory of the atom, now known as Dalton’s Atomic Theory, which consisted of three main ideas:
- All substances are made of atoms. Atoms are the smallest particles of matter. They cannot be divided into smaller particles. They also cannot be created or destroyed.
- All atoms of the same element are alike and have the same mass. Atoms of different elements are different and have different masses.
- Atoms join together to form compounds. A given compound always consists of the same kinds of atoms in the same ratio.
Dalton was remarkably on point, considering the limited scientific tools he had at his disposal to investigate such a grand mystery of the universe. His ideas were quickly embraced and most of them withstood the test of time even to this day, except his principle that atoms are the smallest particle. We now know that there is an entire zoo of subatomic particles, from quarks to bosons, many of which still await discovery.
After Dalton’s brilliant forays into the microcosmos, the next major advance in the history of the atomic model was the discovery of electrons in 1897 by another British scientist named J. J. Thomson. During the late 19th century, it was known that electric charges could either be positive or negative, but it wasn’t clear what these charges were made of.
Thomson conducted a series of experiments in which he sent electric currents through gases inside vacuum tubes. He observed that the gases would give off light, a phenomenon known as cathode rays. He later posited that cathode rays are made of tiny particles he called “corpuscules”, the fundamental building blocks of electric charge.
But Thomson didn’t stop there. He wanted to know more about these corpuscles, so he conducted a series of experiments to measure their properties. He found that they had a negative electric charge, and that their mass was much smaller than that of atoms. In other words, Thomson had discovered the electron. Prior to Thomson, scientists thought that electrical currents consisted of rays, similar to rays of light, and that they were positive rather than negative.
The nature of the electron intrigued but also confused Thomson at first. He knew that electrons had a negative charge but he also had evidence that atoms were neutral in electrical charge. It followed that the rest of the atom must be positively charged to cancel out the electron charge, and used this assumption to devise a new atomic model — and he did this in the most British way imaginable.
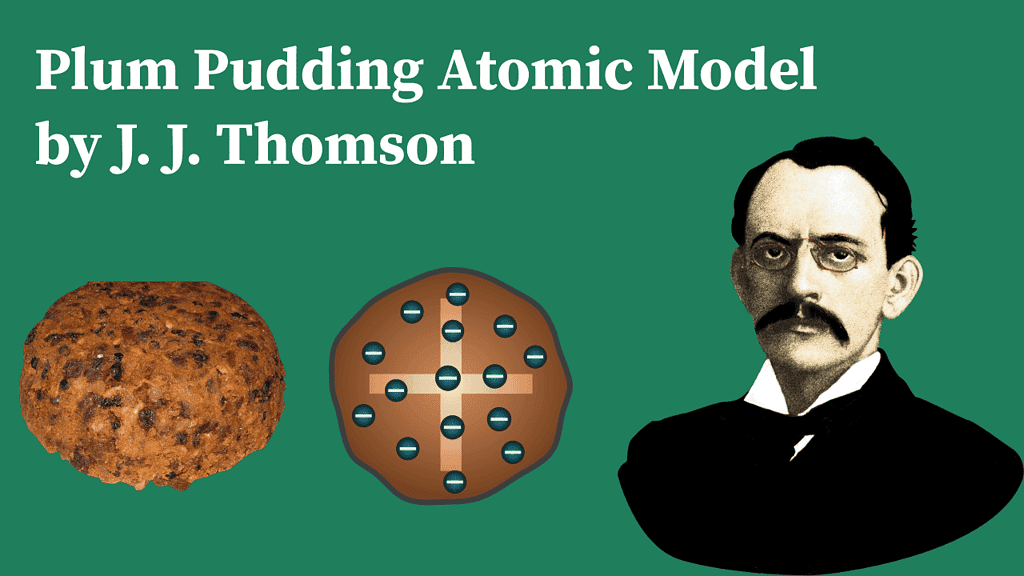
Thomson imagined the atom like a plum pudding, a British dessert that has plums scattered throughout it. In Thomson’s Plum Pudding Model the atom is a sphere of positive charge, the pudding, while the negative electrons, the plums, are scattered throughout it.
Alas, as deliciously simple as it sounded, the Plum Pudding Model was wrong, as another physicist, this time from New Zealand, would show. In 1899, Ernest Rutherford discovered that some elements give off positively charged particles, which he named alpha particles. In 1911, he shot a beam of alpha particles at a very thin sheet of gold foil, with a screen placed on the opposite side of the foil that would glow when alpha particles struck it.
If the atom was shaped like a pudding, the alpha particles should be deflected as they pass through it because the positively charged gold pudding should repel the positive alpha particles. But that didn’t happen. Instead, most of the alpha particles passed straight through the foil as if it was empty space.
Rutherford correctly asserted that positive charge is not evenly spread throughout an atom, but instead is concentrated in a very tiny volume he called the nucleus, which contains positively charged particles he named protons. His student James Chadwick would later confirm the existence of neutrons in 1932. The rest of the atom is virtually empty space except for those pesky electrons that move randomly through it.

Having proven Thomson’s model wrong, it befell Rutherford to come up with an alternative model, in which he compared the random motion of electrons around the atomic nucleus to planets orbiting a star. In 1913, Danish physicist Niels Bohr picked up from where Rutherford left off and refined his model, introducing his quantized shell model of the atom to explain how electrons can have stable orbits around the nucleus.
Bohr modified the Rutherford model by requiring that the electrons move in orbits of fixed size and energy. The energy of an electron depends on the size of the orbit and is lower for smaller orbits.
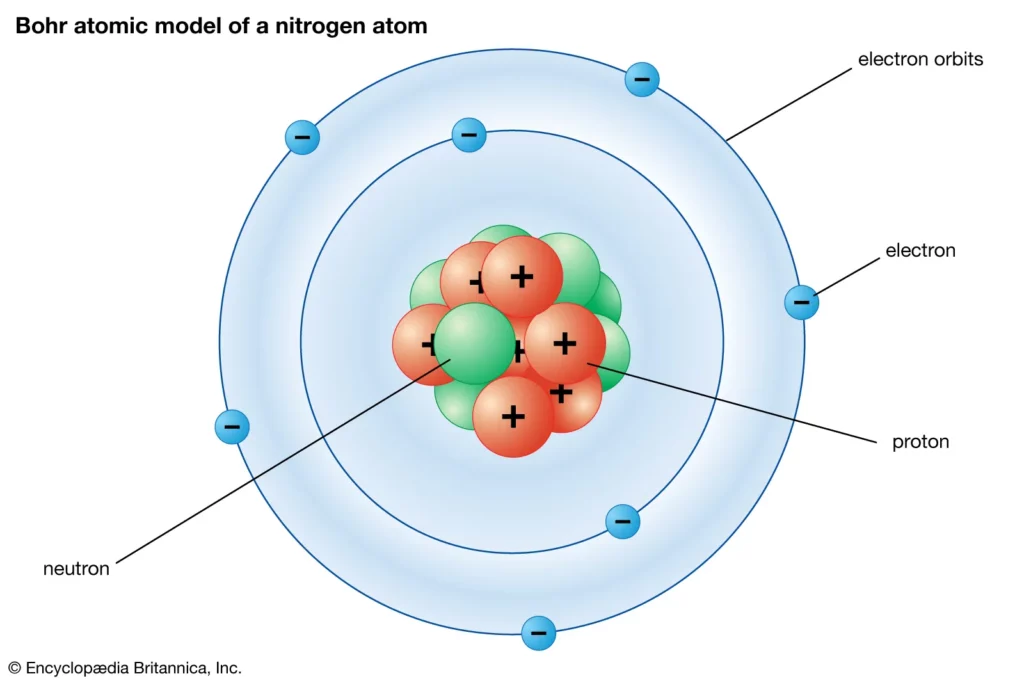
The Rutherford-Bohr atomic model could explain many of the observed properties of atoms and quickly became the dominant view of the atomic structure for decades to come. It seemed like scientists had finally figured out the atom. Except they didn’t. It looks like there was always someone ready to one-up the previously expired atomic model.
Inside the electron cloud: visualizing the probability of an electron’s location

As scientists continued to study the atom, they began to uncover new phenomena that couldn’t be explained by the Bohr model. For example, they observed that atoms could sometimes emit or absorb energy in specific, discrete amounts, a phenomenon known as quantization. This didn’t make sense within the framework of the Bohr model, which treated electrons as smooth, continuous objects.
To explain these strange observations, a new model of the atom was needed. This model would have to take into account the fact that particles, as in quantum entities, can behave both like waves and like particles, a phenomenon known as wave-particle duality. It would also have to account for the uncertainty principle, which states that it is impossible to know both the position and the momentum of a particle with complete precision.
Enter quantum mechanics. This revolutionary new theory, developed in the 1920s and 1930s by physicists like Albert Einstein, Niels Bohr, Max Planck, and others, provided a new way of thinking about the atom. Instead of treating electrons as particles with definite positions and velocities, quantum mechanics treated them as probability waves, spread out over a range of possible positions.
All of this new physics culminated in the electron cloud model proposed by Austrian physicist Erwin Schrödinger in 1926. He defined the electron cloud as a region where electrons form a three-dimensional standing wave. Schrödinger used mathematical equations to describe the likelihood of finding an electron in a certain position, including his famous Schrödinger’s equation that describes how the quantum state of a quantum system changes with time. The probability function describes a cloud-like region where a particular electron is likely to be found — but never with certainty — hence the name.
This so-called “electron cloud” model of the atom was able to explain the observed properties of atoms much more accurately than the Bohr model. It also allowed scientists to make predictions about the behavior of atoms with unprecedented precision. The move from the Bohr model of the atom to the electron cloud in quantum mechanics was a major milestone in our understanding of the universe at the smallest scales. It allowed scientists to make sense of strange and counterintuitive phenomena, and it has had a profound impact on the world we live in today.
The Role of the Electron Cloud in Atomic Structure
The electron cloud plays a crucial role in the way that atoms are structured. The arrangement of electrons in an atom determines many of the chemical properties of the element, such as its reactivity and its behavior in different chemical reactions.
The electron cloud also plays a role in the way that atoms bond with each other. When two atoms bond together to form a molecule, the electron clouds of the two atoms overlap, creating a region of space where the probability of finding an electron is higher than it is in the individual atoms. This overlapping of electron clouds is what gives molecules their characteristic shapes and properties.
Overall, the electron cloud model has greatly expanded our knowledge of the fundamental nature of atoms and molecules, and has opened up many new areas of research in both physics and chemistry. It continues to be an important concept in our understanding of the building blocks of matter and their behavior.
Was this helpful?