There is more than one type of liquid water out in the universe, researchers explain.

We’re all familiar with liquid water here on Earth. But in the high-pressure, low-temperature conditions present in far-flung areas of the universe, liquid water may look and behave in completely unexpected ways. At least, that is the conclusion of a collaboration between theoretical physicists at the University of Birmingham in the UK and Sapienza Università di Roma in Italy.
By studying the behavior of water molecules placed under high pressure in temperature ranges that would usually cause the liquid to crystallize, the team identified the key features of two new liquid states of water.
Shapes of water
When placed under high pressure at low temperature, the team explains, the H2O molecule takes on an unexpected shape: it ties itself into knots. This shift allows it to remain liquid even under conditions that would normally see it crystallizing into ice.
This research expands on theoretical work laid down in the 1990s that attempted to determine what interactions take place between supercooled water molecules — molecules that are taken below their typical freezing point without solidifying.
Researchers have been steadily pushing the limits of supercooling water for a few years now. The current-lowest temperature they have been able to reach while keeping water liquid is –263 degrees Celsius (–441 degrees Fahrenheit), although this feat can only be performed for moments at a time.
Although we’ve come so far in maintaining liquid water in a supercooled state, we were not any closer to understanding exactly how their molecules are behaving.
What we could infer is that, when water molecules are drained of heat (which, on a molecular level, looks like disorderly motion), their competing polar attraction forces must push them into taking on new configurations. But actually observing them in the supercooled state is still beyond our capabilities — so the team resorted to computer simulations instead.
Since the actual behavior of a material is extremely complicated, the team simplified their simulations to only take into account significant variables. In this case, they modeled ‘clumps’ of water as if they were larger particles suspended in the liquid, instead of individual molecules. This allowed them to get a better understanding of how groups of molecules interact and how they change from one arrangement to another.
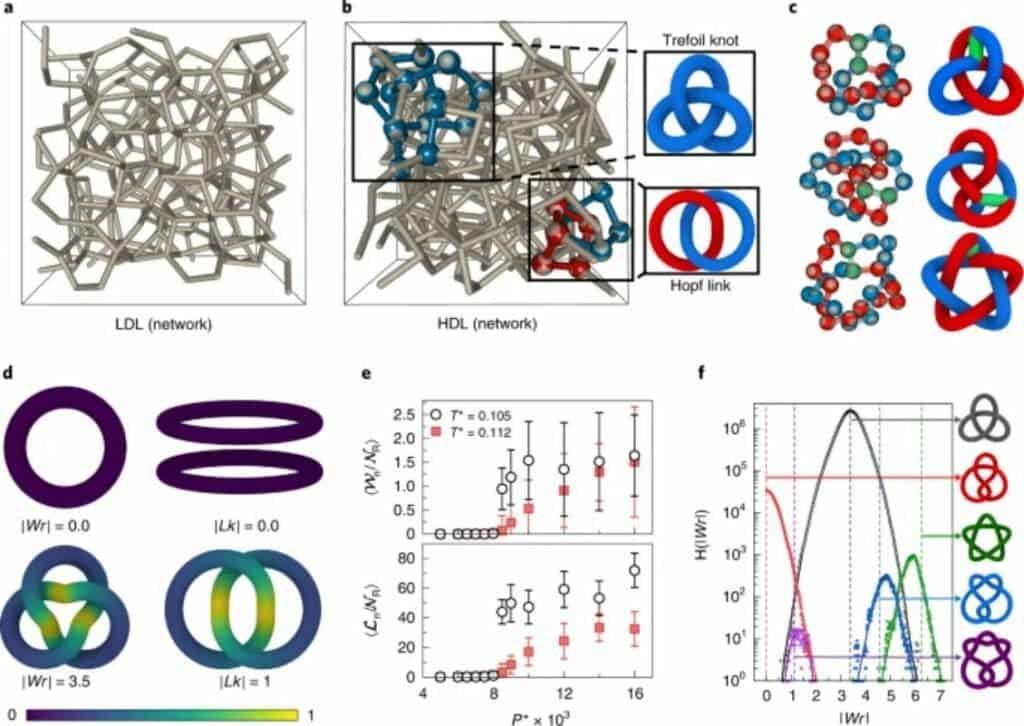
The simulations based on this model showed that at one point in the supercooling process, water molecules switch from pushing apart to pulling on each other, settling closer to one another and creating a denser liquid. The shape of molecular interactions (‘topology’) in this state also looks completely different from those in water at room temperatures, the team adds. Molecules become entangled in intricate networks while pulling in on each other, and much simpler arrangements once they start pushing apart again. The first or ‘topologically complex’ state is similar to a pretzel in shape, with the molecule tied into an overhand knot. The second one is a simpler series of rings.
“This colloidal model of water provides a magnifying glass into molecular water, and enables us to unravel the secrets of water concerning the tale of two liquids,” says University of Birmingham chemist and paper co-author, Dwaipayan Chakrabarti.
“I am sure this work will inspire novel theoretical modeling based on topological concepts,” says Francesco Sciortino, a condensed matter physicist at Sapienza Università di Roma, corresponding author of the paper.
These structures are reminiscent of the long chains of covalent bonds seen in some organic compounds.Unlike these bonds, however, these ‘knots’ are short-lived, the team explains. But, judging from their structure, the nature of liquid water in high-pressure, low-temperature environments should be radically different from what we know of on Earth’s surface.
A better understanding of the topological behavior of supercooled liquids could go a long way into helping us prepare for worlds with extreme environments. If we don’t understand how different materials will behave under those conditions, our explorers might find themselves unprepared for tricky — or deadly — situations.
The paper “Topological nature of the liquid–liquid phase transition in tetrahedral liquids” has been published in the journal Nature Physics.
Was this helpful?