Time crystals exhibit a periodic structure across time and promise to provide valuable insight into quantum systems. Up until recently, this exotic state of matter was thought to exist in the theoretical realm only. Some physicists have gone as far as claiming they’re impossible to reproduce in the real world. But a group from Harvard University defied the impossible and showed how to build one. It turns out, you need to eventually give it a good wack.
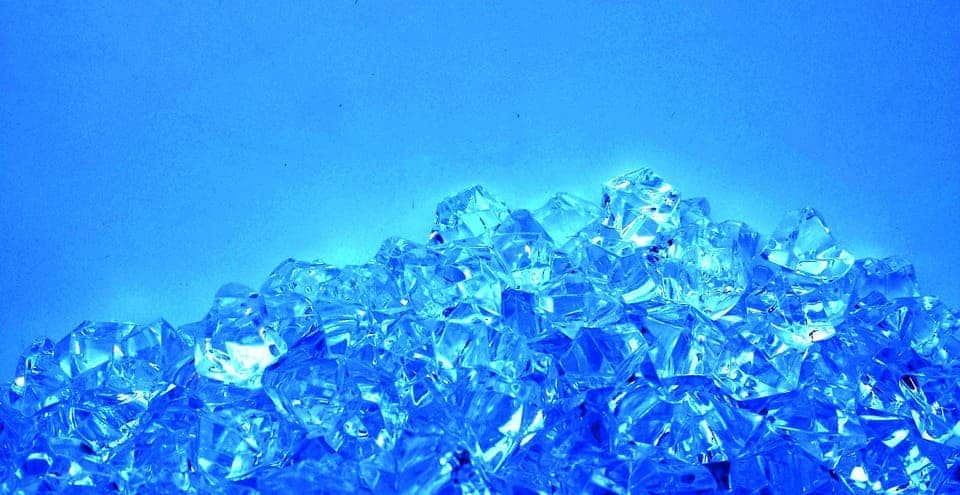
Repeating in time
Whether its salt or quartz, a crystal is defined as the periodic arrangement of atoms in a 3-D lattice. Glass, wax, or plastics, on the other hand, have no period structure whatsoever — they’re called amorphous solids.
Time crystals are essentially regular, boringly ordered crystals with a twist. A fourth dimension, time, is added so the material exhibits different periodic structures over time. What makes these crystals particularly remarkable is less that they repeat in time but rather has more to do with the fact that they’re intrinsically out of equilibrium. Because time crystals are never able to settle down, say into a diamond or ruby, there’s a lot we can learn from them.
“There is now broad, ongoing work to understand the physics of non-equilibrium quantum systems,” said Harvard Professors of Physics Mikhail Lukin in a statement. “This is an area that is of interest for many quantum technologies, because a quantum computer is basically a quantum system that’s far away from equilibrium. It’s very much at the frontier of research…and we are really just scratching the surface.”
Time crystals were first proposed in 2012 by Nobel laureate Frank Wilczek and ever since scientists have experimented to prove their existence. Initially, the notion that a time crystal could actually be built seemed unlikely. Some have gone so far as to prove that it would be impossible to create a time crystal in a quantum system that was at equilibrium.
Sure, most systems are at equilibrium — but not all of them. Earlier this year, the first time crystals were made not by one, but two groups who had worked independently. The first to report their findings were physicists from the University of Maryland who based their material on a blueprint proposed by Norman Yao, an assistant professor of physics at UC Berkeley. The other group is from Harvard University which also worked with Yao’s blueprint but with a different setup from that used at U of Maryland.
The Harvard time crystal naturally started with a material that is out of equilibrium — a diamond. At the same time, the diamond is meta-stable since once it adopts a crystal formation, i.e. after the heat and pressure that went to form it are removed, it will stay in that configuration. Before time crystals were first made in the lab, previous research showed that non-equilibrium systems, sometimes called ‘driven’ systems, share some of the characteristics of a time crystal. One such characteristic is that the crystal remains robust across time with respect to perturbations.
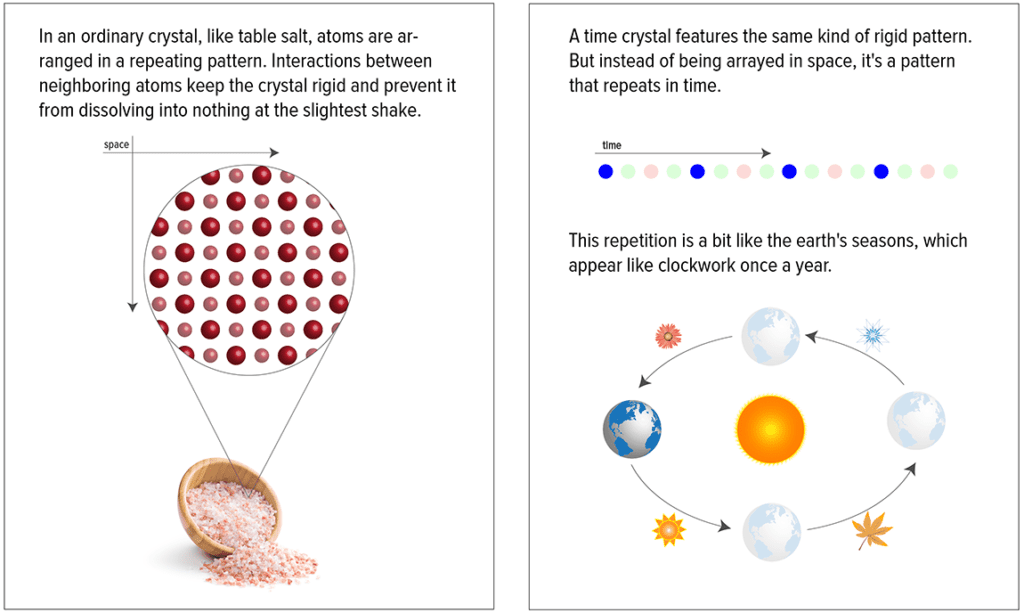
“A solid crystal is rigid…so if you push on it, maybe the distance between atoms changes a little, but the crystal itself survives,” he said. “The idea of a time crystal is to have that type of order in a time domain, but it must be robust.”
Lukin and colleagues first embedded a diamond with millions of atomic-scale impurities known as nitrogen-vacancy (NV) centers. The impurities were so numerous that the diamond became black. The diamond was then subjected to microwave pulses which changed the orientation of the spins of the NV centers. As a pulse changed the spin of the atom from up to down, a subsequent pulse turned the spin back up.
If all the spins don’t fully change from up or down, you get a completely random system. But this isn’t the case because the NV centers stabilize the response, forcing the system to respond periodically. Basically, because the atoms repeatedly change their spin and the spins interact, the atoms can’t settle into a stable, repetitive pattern. This is our time crystal.
So what is it good for?
Time crystals could become critical components in quantum computers and quantum sensors because these can support long quantum memory times and a very high density of quantum bits.
“For many applications you want both of those,” Lukin said. “But these two requirements are usually contradictory….this is a well-known problem. The present work shows that we can achieve the desired combination. There is still a lot of work to be done, but we believe these effects might enable us to create a new generation of quantum sensors, and could possibly in the long run have other applications to things like atomic clocks.”
There are also more immediate applications for time crystals.
“One specific area where we think this might be useful, and this was one of our original motivations for this work, is in precision measurement,” Lukin said. “It turns out, if you are trying to build…for example, a magnetic field sensor, you can use NV-center spins,” he said. “So it’s possible these non-equilibrium states of matter which we create may turn out to be useful.”
Journal reference: Soonwon Choi, Joonhee Choi, Renate Landig, Georg Kucsko, Hengyun Zhou, Junichi Isoya, Fedor Jelezko, Shinobu Onoda, Hitoshi Sumiya, Vedika Khemani, Curt von Keyserlingk, Norman Y. Yao, Eugene Demler, Mikhail D. Lukin. Observation of discrete time-crystalline order in a disordered dipolar many-body system. Nature, 2017; 543 (7644): 221 DOI: 10.1038/nature21426.
Was this helpful?