New research is in hot pursuit of life’s first energy-producing reactions.
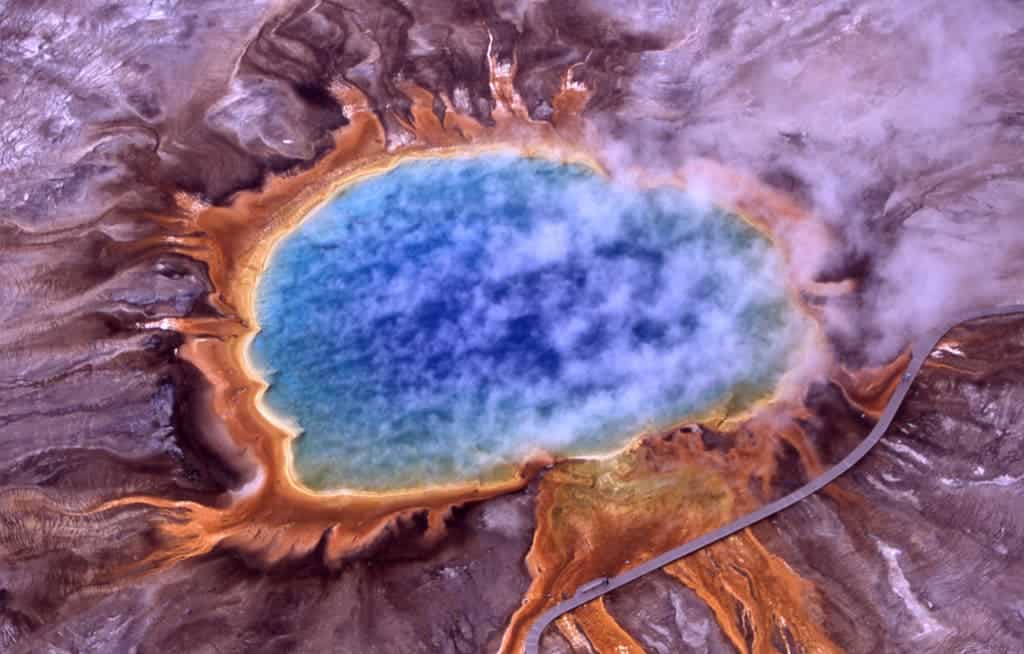
What’s life worth if one can’t enjoy a good meal, right? Or, failing that, any meal at all? Not very much, since our bodies need to turn that food into fuel to keep going — and they have very sophisticated mechanisms on hand to do just that.
Our cells turn oxygen organic compounds, especially sugars, into energy and CO2 through a process known as cellular respiration. You may have been told in school that mitochondria, the specialized organelles which handle cellular respiration, ‘burn’ food to release energy, and that’s a pretty functional illustration of the process. The whole picture is, however, much larger that.
Energy-producing mechanisms in our body are often referred to as cycles — one of the most common ones is the citric acid / Krebs cycle. These are usually very complicated processes, and as such, are very difficult to wrap your head around and study them in the lab. Which is a pain if you’re the kind of scientist who’s trying to understand how these cycles came to be, where they first started from, and how they evolved. However, new research could offer these researchers the lucky break they need.
A team led by Greg Springsteen, Professor at the Chemistry Department of Furman University, South Carolina, has identified what seems to be an ancient precursor of the citric acid cycle, one that could exist in the much less-refined conditions of early Earth and sustained early life.
“It’s akin to looking at a modern roadway like Route 66,” Springsteen, who’s also affiliated with NASA Center for Chemical Evolution, Atlanta, explains.
“Wagon trails were templates for Route 66. The twists and turns of the modern pathways tell us about the challenges of the original route.”
The Krebs cycle works with a chemical known as pyruvate through a series of reactions over which the molecule changes its form (through subsequent addition/removal of atoms and molecules). The end results are energy and chemical bits and pieces to be used in other reactions throughout the body. Since organisms need a lot of energy, the Krebs cycle is propped up by specially-designed enzymes which make every step in the reaction go buttery-smooth. Because of all these extras pitching in, it can be hard to figure out how the cycle looked initially, and what chemicals could have sustained the process before the enzymes came along.

However, the team, which also included members from the Scripps Research Institute in California and two undergrads at Furman, found two compounds which could maintain a Krebs-like cycle in experiments mimicking conditions on early Earth. Christened the HKG- and malonate-cycles, the team says these processes are likely very similar to the pre-life versions of the reactions that keep us alive today.
They’re hugely less efficient than the Krebs cycle, but that was to be expected, given the lack of supportive enzymes. More importantly, however, they’re based on a similar chemical blueprint — both cycles turn a molecule called glyoxylate into CO2 and other molecules in the presence of an electron-capturing agent. They’re so similar, the authors’ hypothesis is that biology picked them up as it was and simply tweaked and improved upon them, leading to the reactionary cycles we see today.
“We think biology mapped itself onto preexisting chemical pathways on the early Earth,” said Springsteen.
As exciting as the findings may be, take them with a grain of salt. The team doesn’t yet have much to offer in regards to how the cycles evolved over time into today’s Krebs’, and they’re yet to find the reactions occurring naturally or to prove that they did so before life took hold on Earth. Still, the two new cycles have a lot going for them — the reactions occur in a neutral (not acidic or basic) environment and in a mild temperature range. “There were environments on the early Earth where these reactions were reasonable,” the authors note.
At the very least, they give us a possible starting point from which to expand our search.
The paper “Linked cycles of oxidative decarboxylation of glyoxylate as protometabolic analogs of the citric acid cycle” has been published in the journal Nature.